Generation and
Transmission Investments in Restructured
Electricity Markets
Rajat K. Deb, Pushkar Wagle, and Rafael Emmanuel
A. Macatangay §
Abstract
The efficient
expansion of generation and transmission facilities is one of the biggest
challenges in restructured electricity markets. Competition has become the
mechanism for encouraging generator entry and transmission investment. Market
decisions on generation and transmission investments are separately made but
affect each other intimately. The impact of generator
entry, depending on size, location, and timing, on a power system is different
to that of transmission investments. Indeed generator entry outside a region
could simultaneously increase congestion on an interface connecting to that
region and reduce congestion costs in the entire region. Market players
need to understand and model the complex interaction between generation and
transmission investment. As a demonstration of the complexity of the modeling
challenges, UPLAN, a proprietary engineering economy model of the
North American power system, is used to forecast unserved energy and congestion
costs in the WSCC for 2005. UPLAN rigorously accounts for merchant plant and
transmission line additions as well as for the fundamental drivers of WSCC
power markets.
Outline
- Generation, transmission, and system
reliability in restructured electricity markets
- Market signals for investments in generation
and transmission
- Analyzing and modeling investments in
generation and transmission
- The impact of generation and transmission
investments on unserved energy and congestion costs
1. Generation,
transmission, and system reliability in restructured electricity markets
The
nature of the interaction between generation and transmission has been
considerably altered under restructuring. In the days of the vertically
integrated utility, a single firm made planning decisions on both generation
and transmission and, as a consequence, internalized most of the externalities
associated with them. The traditional planning process for generation was
focused on a particular index of reliability, usually the loss-of-load
probability (LOLP), in all planning years and for a given minimum revenue
requirement. The logic was quite straightforward: transmission expansion
improves reliability and reduces both LOLP as well as the need for generation
investment. The reduction in LOLP depends on the size of the new line as well
as on the correlation of the loads in either end. The optimal amount of
generation investment, therefore, can be calculated, given alternative line
investments and with the use of LOLP as a criterion for generation expansion.
Thus,
in the traditional planning world, generation and transmission were
substitutes, and the substitution decision was made by the vertically
integrated utility. Generation and transmission expansions were designed to
minimize the total discounted cost of delivering uninterrupted power to the
utility’s customers. At each point in the grid, in every hour, and for every
reasonable contingency, generating capacity has to be sufficient to meet loads.
When the grid is at capacity, however, some loads can be served only by
increasing the output of local generation. The planning process aims to
compare, given an LOLP target, the cost of delivering new generation to the
cost of reinforcing the grid.
A
number of models were routinely used to perform reliability analysis. The PICES
model, developed at the Oakridge National Laboratory, uses equipment failure
rates and specified reserve levels in order to calculate an optimal generation
expansion plan. If the LOLP target is not reached, then the reserve-margin
criterion may be raised. EGEAS, developed by the Electric Power Research
Institute (EPRI), and WASP, developed by General Electric (GE), enables
a utility to develop an optimal generation expansion plan with a suitable
reserve margin in place (such that LOLP targets are met in each year of the
planning period). The “Over/Under” Capacity Model, developed by EPRI in the
early 1980s, evaluates the trade-off between reserve capacity and reliability.
Today, by
contrast, the restructuring of the electric power industry has caused the
unbundling of generation, transmission, distribution, and supply (or retail
sales). Markets govern the operation and expansion of generation and
transmission, which have ceased to be perfect substitutes in a world of
competition. Dispersed players separately make decisions that collectively
affect system adequacy. Potential generation investors are interested in a
location’s energy prices that, in future, are influenced by changes in the
transmission system. The profit opportunities arising from transmission
reinforcements are affected significantly by the location of generator entry.
New generation may decrease congestion in certain parts of the system but may
increase it in others, and new transmission may decrease the use of already
under-utilized lines. Thus, generation and transmission investments alter each
other’s revenue expectations.
Most strikingly, LOLP has become a rather limited
measure of reliability. It accounts only for generator outages, assumes no
uncertainty in demand, and is, by nature, long-term. Under a restructured
environment, however, merchant plant additions are driven by commercial
considerations, and capacity additions are not dictated solely by reliability
measures. In short, in the restructured power industry, markets, not mavens,
rule. Clearly a modern approach to reliability analysis has to be based firmly
not only on the physics of electric power systems but also on the economics of
restructured energy markets.
2. Market
signals for investments in generation and transmission
Under
a restructured regime, the appropriate size, timing, and location of generation
and transmission investments typically depend on increasingly complex market
interactions. For example, reserves in one area can be used to serve another.
As a consequence, energy prices could be lower than otherwise, and investments
in either generation or transmission could be postponed. The impact of
generator entry, depending on size, location, and timing, on a power system is
different to that of transmission investment.
The
market signals for generation and transmission investment are diverse
and
continue to evolve. A plant could make money from day-ahead and
hour-ahead
markets for energy and ancillary services. Price duration and
fluctuation have
important profit implications (see Figure 1). Maximum profits are
achieved from
an optimal allocation of a plant’s output to the various
cascading markets for
power, as well as from correctly bidding a plant’s opportunity
cost (the
greater of marginal production cost and the expected prices in the
different
market). In some cases, depending on the market rules for reserve
capacity
payments, a plant might earn more from ancillary services than from
energy. One
of the most crucial market signals is in relation to reliability and
reserve
sharing. Different regions in North America have taken innovative
steps. For
example, in the restructured electricity systems of the Northeast (New
York, PJM, Ontario, and New England), a market has been created
specifically for
long-term capacity. Load-serving entities can purchase reserves in
order to
meet their responsibility of carrying monthly reserves. The product in
these
monthly reserve markets is designed as six-month strips of capacity
recently
selling for $10/kW to $18/kW.
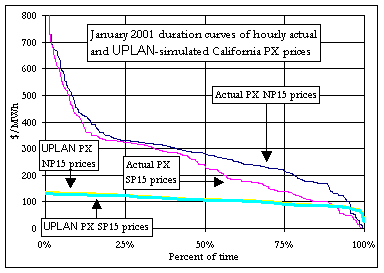
In
addition to long-term capacity and reliability, New York, PJM, New
England, and California ISO operate and manage markets for ancillary
services, such as
regulation, spin, and non-spin reserves, on a day-ahead basis.
Sophisticated
protocols have been developed to encourage participation as well as to
regulate
prices. In California, the ISO maintains reliability must-run reserve
(RMR)
contracts to support reserve margin requirements in
generation-deficient but
transmission-constrained load-pockets. Texas has created an ancillary
services
market and is now doing trial runs. In the rest of the US, however, the
capacity market for reliability is not fully developed. There is no
open
market for capacity, although bilateral deals are quite common.
Reliability and
capacity can also be provided through financial instruments. For
example, Enron
uses options on capacity and weather as a way of managing the risk of
shortfalls in capacity and reliability. Finally, in other markets,
reliability
is indirectly traded as firm and non-firm contracts.
The
emergence of regional transmission organizations (RTOs) considerably increases
the need for close coordination between generation and transmission expansions.
As described in FERC Order 2000, which is on the functions of an RTO, the
reliability issue is no longer a generation issue alone and now includes the
impact of both generation and transmission on a wider area than individual
utility service territories. The benefits and costs of carrying reserve are
distributed across service territories due to network effects. Both federal and
state commissions are to develop new protocols for allocating the costs and
benefits of reserve margins over dispersed regions. Indeed RTO tariff design
affects a merchant generator’s response to load growth in the context of an
evolving transmission system. Moreover, periodic shortages in generation
typically lead to episodes of market power exercise. The RTO has to contend
with the usual results, such as severe and clustered price spikes, inefficiencies
in grid operations, and violations of merit-order dispatch.
Finally,
transmission expansion is closely related to the growth of loads and resources,
but its financing depends on expected congestion and congestion revenues.
Transmission expansion, therefore, has to be balanced with generation
expansion, given that generator location affects congestion revenues. One of
the most important signals for transmission investment is the accumulation of
congestion rent, which is a line’s economic value estimated as the difference
in energy prices between one end and another. High congestion costs usually
signal the need for grid expansion. However, expansions that eliminate those
rents would also remove much of the market basis of the expansion in the first place.
Eliminating congestion through transmission expansion would require the
imposition of usage fees on all generation and load, with no locational price
signal. Some grid expansions may remove the need for certain site-specific
generation. In the end, the plant fleet could have few and large units (and
quite possibly be more efficient than one that has many and small ones).
One
key consideration is volatility. The factors influencing the generator entry
decision, such as energy and ancillary service prices, load, economic growth,
fuel prices, and emission allowance prices, are volatile, and their volatility
has to be analyzed rigorously. The factors influencing transmission investments
are very similar and thus also volatile. Another key consideration in the
decision to invest in transmission lines is the difficulty of acquiring the
required planning and related permits. A new plant is to be situated in only
one spot, and the markets for obtaining fuel contracts and emission allowances
are well developed. By contrast, a new transmission line potentially straddles
many areas, and there is usually a huge outcry over the replacement of rolling
hills and lush landscapes with monstrous transmission pylons. The direct and
indirect costs of compensating stakeholders for the perceived loss of
countryside beauty are another form of “market” signal that transmission
investors have to receive.
3. Analyzing
and modeling investments in generation and transmission
In a
restructured electricity industry, the market realities faced by generation and
transmission investors are exceedingly complex. Price is determined by a
confluence of a large number of different events and factors (see Figure 2).
Supply and demand are just one set of factors. The status of deregulation and
restructuring, including the design of market rules, is a major determinant of
prices. All possible market design loopholes and legal inconsistencies are
exploited for profit. The physical features of the grid are also crucial. The
status-quo pattern of transmission constraints is usually beneficial to some
generation and transmission owners but detrimental to others. Any proposed
alteration of the network implies a redistribution of rents, a change in
bidding strategies, and, depending on the location of the players, a
realignment of alliances. The interaction of the different markets influences
capacity deployment. The potential for earning capacity payments in the
ancillary service markets is a powerful incentive to withdraw capacity from the
energy market, in which payments are purely on energy. Climactic factors are
especially important for hydro units. Expectations of drought and unfavorable
changes in weather patterns increase the scarcity value of water and worsen any
strategic behavior exercised by the hydro unit. Thus, many interacting factors
are at work, and any analysis of generation and transmission investment quickly
becomes intractable.
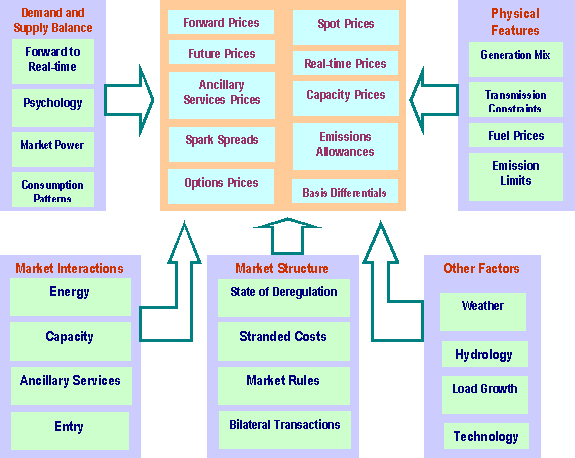
Sophisticated
analysis and modeling are evidently needed. The first analytical challenge is
an accurate representation of the various markets and the bidding behavior they
encourage. Profits are made in energy and ancillary service markets clearing
day-ahead, hour-ahead, and in real-time. In maximizing profits, therefore, a
plant has to bid its true opportunity cost, which is the greater of its
marginal production cost and the expected prices in the different product,
temporal, and spatial markets. Another related factor is the expectations of players.
Market participants are typically well informed: many of the executives are
carry-overs from the days of vertical integration, the technology of power
plants is fairly well known, high quality data on the industry are abundantly
available, and most importantly, frequent market interaction, on an hourly
basis in many markets, facilitates learning. Bidders can be said to have an
intimate understanding of the underlying factors driving market outcomes.
A
second analytical challenge concerns the locational dimension. The value of
energy is different across the power system, and an accurate representation of
the physics of power systems is thus quite central. An alternating current (AC)
representation of the system is a detailed and accurate picture of the
transmission lines, their links to one another, and the flow of power in and
around them. For example, an optimal power flow (OPF) is a traditional
engineering tool for analyzing the dispatch of plants in order to meet loads
across the system. An AC OPF could be used to evaluate two alternative
investments, one in generation and another in transmission, and determine how
they affect current and future prices across the network and for different
products.
A
third and final analytical challenge is the inherent uncertainty in complex
phenomena. Electricity markets are subject to severe fluctuations in fuel
prices (such as oil and natural gas), emission allowance prices (for SOx
and NOx), and regulatory interventions. Volatility analysis is
crucial to the avoidance of costly investment mistakes. Moreover, investments
in generation (and to some extent, transmission) are flexible and, in the event
new information is obtained, can be postponed. A real options approach to
investment under uncertainty has to be deployed in order to take advantage of
the plant’s flexibility and acquisition of fresh commercial information.
In
summary, the impact of generation and transmission investments on power prices
is not easy to assess, and a large number of factors have to be taken into
account. Only specialist models, such as an engineering economy representation
of an electric power system, can do a proper job of capturing the key market,
physical, regulatory, and climactic drivers underlying market outcomes.
4. The impact of generation and transmission
investments on unserved energy and congestion costs
The impact of
generator entry and transmission expansion on unserved energy and congestion
costs in California and the WSCC is forecast using UPLAN,
a proprietary engineering economy model (see Figure 3). UPLAN
satisfies the steep requirements discussed in the previous section. It has four
key components: a market model, an AC OPF, a volatility model, and a merchant
plant model. The market model captures the multi-market multi-commodity nature
of a restructured power industry. It utilizes opportunity cost bidding and thus
captures the optimization behavior of a generator across product, temporal, and
locational markets. The AC OPF is a faithful representation of the physical features
of the grid. It is accurate for each bus and line in the system. The volatility
model allows the calculation of the real option value of the plant for a
distribution of market outcomes. It is a powerful approach to the evaluation of
risk. Finally, the merchant plant model is a dynamic model of generator entry.
Generator investment decisions are endogenously determined by the profit a
potential entrant is expected to earn. UPLAN employs a rational expectations approach to the
behavior of players: solutions are obtained iteratively, and as a consequence,
the information set of each player consists of the underlying determinants of
market outcomes. UPLAN, therefore, is a mathematical replica of the US power market. [2]
The analysis of generation and transmission
adequacy[3]
consists of a three-step simulation of the system: first, a base case, before
any generation addition in future years; next, an optimal expansion of
generation and transmission; and finally, an analysis of adequacy given the
plant additions. The simulation resulted in the addition of about 3,500 MW of
capacity at different locations in the WSCC (but all outside California) in
2004 and 2005.
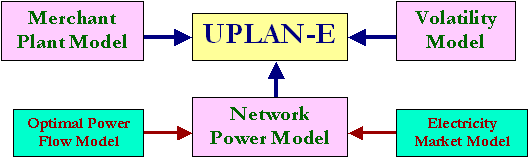
Figure 3. Schematic of UPLAN
Generator
entry reduces the level and frequency of unserved energy in the WSCC (see
Figure 4). However, there is no significant difference in the flow duration
curves[4] for
the California-Oregon Interface (COI) and the Path 15 interfaces before and
after generation expansion (see Figure 5). All the units added in the
simulation are outside California, and thus they do not have a significant
impact on the interface between California and Oregon.
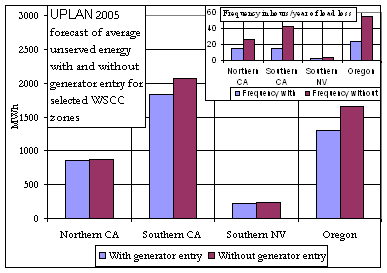
Figure 5. Flow Duration Curves With and Without
Generation Expansion for WSCC, 2005
The duration and marginal and total annual costs of
congestion on selected transmission interfaces in California, given the
simulated generation and loads for 2005, are in Table 1. These congestion costs
represent the differences, net of the relevant grid-access or wheeling charges,
between locational prices on either side of the interface, over the hours
during which the interface is constrained. They are, therefore, the marginal
value of additional generation. If transmission capacity is added, then the
congestion will disappear, and owners of new lines would have to recover their
investment through appropriate transmission charges.
Table 1. Transmission
Adequacy and Congestion Cost at Selected Interfaces in the WSCC, 2005
(a) After unit addition
Interfaces
|
Zones
|
Average % of Congested Hours
|
Average number of Congested
Hours
|
Std Dev of Hours Congested
|
Total Congestion Cost ($)
|
Std Dev of Total Congestion
Cost
|
Average Congestion Cost ($/KW)
|
Std Dev of Average Congestion
Cost
|
|
|
COI
|
Oregon
|
Northern California
|
2.09
|
183.18
|
178.66
|
91.33
|
86.87
|
0.03
|
0.03
|
|
PATH15
|
Central California
|
Northern California
|
7.77
|
680.55
|
977.58
|
571.81
|
1029.02
|
0.21
|
0.38
|
|
(b) Before unit addition
Interfaces
|
Average % of Congested Hours
|
Average number of Congested
Hours
|
Std Dev of Hours Congested
|
Total Congestion Cost ($)
|
Std Dev of Total Congestion
Cost
|
Average Congestion Cost ($/KW)
|
Std Dev of Average Congestion
Cost
|
COI
|
0.56
|
48.86
|
336.79
|
54.33
|
481.46
|
0.01
|
0.13
|
PATH15
|
1.12
|
97.84
|
440.03
|
88.47
|
514.35
|
0.03
|
0.19
|
The
situation for generation investors is quite different. The addition of
generating plants increases congestion costs on the COI interface.[5]
On average, the COI interface is congested for 183 hours with generation
expansion (see Table 1a), but for only 49 hours without (see Table 1b).
Generator entrants have shifted the supply curve outwards, and as a result, at
the same price, a greater quantity of power can be exported than without
generator entry. However, system-wide congestion costs are lower with than
without generation expansion (see Figure 6). The additional generation, which
in principle is cheaper than existing units, has decreased the price difference
between one end of the interface and the other. Thus, generation investments
outside California reduce not only the level and frequency of unserved energy
in California but also average and total congestion costs in the WSCC.
Figure 6. Duration Curves of Projected Congestion
Cost With and Without Generation Expansion in the WSCC,
2005
In
summary, generation and transmission investments have different effects on the
system, and the incentives motivating them are also different. In the
simulation, generator entry is most profitable outside California, but the
reduction in WSCC congestion costs and unserved energy, two region-wide
externalities, has not removed the incentive to invest in generation. By
contrast, additions to transmission capacity eliminate the congestion rent and,
probably with them, the incentive to invest in new lines, unless appropriate
transmission charges are designed.