VALUING TRANSMISSION INVESTMENTS: THE BIG PICTURE AND THE DETAILS MATTER - - and Benefits Might Exceed
Expectations
Dr. Rajat Deb and Dr. Keith White, LCG Consulting
Background
The wave of concern provoked by the summer of 2003’s massive
power blackout has largely receded, but in its wake deeply-rooted transmission
investment dilemmas remain. With deregulation and expansion of electricity
markets, the operation and planning of transmission have increasingly become separated
from ownership of generation, and from the retailing function. Besides complicating
transmission planning, this increases the diversity of stakeholder interests, and
accentuates the “who benefits?” question.
Like highways and other infrastructure, electric
transmission is critical to efficient and reliable delivery of services, but would
likely be inadequately funded if left to individual market participants.[1]
One problem is that potential beneficiaries of transmission investments are
numerous and diverse, and may not be located directly on a particular “route” being
funded. Also, benefits will be long-term, influenced by uncertain future
developments including high-consequence outcomes that are individually unlikely.
Finally, it may be inefficient, undesirable and even impossible to control
distribution of benefits.
It is thus essential to value transmission investments from
a network- and market-wide “big picture” perspective of sufficient scope to
capture major consequences and uncertainties. At the same time, assessment of
benefits must be sufficiently detailed and disaggregated to provide an explicit
(if probabilistic) view of how different stakeholders would be affected. This
provides a basis not only for evaluating and negotiating transmission
investments, but also for verifying the credibility of projected benefits.
The “big picture” generally involves “societal value,” reflecting
combined impacts on consumers, producers and recipients of congestion revenues
(discussed below). However, the appropriate measure of value can be ambiguous
or contentious. How far a field should we look geographically? Should we
exclude monopoly (noncompetitive) rents? Should we only consider consumer
benefits, especially where transmission costs are ultimately rolled into
overall rates? We could measure value in terms of reduction in production costs
due to more efficient generator utilization, but this ignores how much consumers
pay above production costs and who receives the payments. How benefits are
distributed among stakeholders may be of considerable importance, especially if
some stakeholders experience large benefits or disbenefits.
In any event, valuation of transmission investments must consider
the fundamental dynamics and integration of transmission networks with
electricity markets. A realistic and sufficiently broad-scoped assessment can capture
benefits that might otherwise be missed or understated, and can facilitate
recognition of stakeholder impacts.
Besides economic efficiency benefits it is necessary to
consider reliability benefits, which requires addressing network
characteristics and operations with sufficient realism and detail. Reliability
may be treated as a threshold issue (it must be good enough, anything better
has no extra value), converted to a monetary value, or left in physical terms
such as loss of load probability. Comprehensiveness and consistency of the
transmission investment valuation process are enhanced by analyzing reliability
and economic benefits in a single integrated methodology. Reliability considerations
can restrict economic operation of generators, while on the other hand, generator
operating and investment strategies can impact reliability.
Reliability and economic benefits sought through transmission
investment may be achieved by alternative means, including new generation of
the right type and location, better use of existing generation, or demand-side
measures. For example, better use of synchronized reserves could help address
reliability problems exemplified by the massive 2003 blackout.[2]
Often the appropriate alternative against which to value transmission
investment is not doing nothing, but rather investing in generation or demand
side measures.[3]
Thus, the transmission investment valuation process must ultimately be able to
address, within a consistent framework, both transmission and non-transmission
alternatives. This requires analytic tools that are sufficiently realistic and
comprehensive in treating the interaction of transmission, generation and loads.
Transmission Investment Valuation: An Example
In the
following example, LCG Consulting’s UPLAN Network Power Model was used to
simulate hourly optimal commitment and dispatch of a simple 6-generator system
serving load over a 7-line transmission network (Figure 1), before and after
upgrading the transfer capability of the line identified as “E2D” from 200 MW
to 400 MW. Value was calculated over one 8760-hour year of operation, from the
perspective of different stakeholder classes, and as well as individual market
participants within classes. The example demonstrates how the interdependent nature
of a simulated AC network obeying electrical principles gives rise to outcomes
that may be counterintuitive, but in which consumers broadly benefit from
network upgrades, especially if the supply system may become stressed in the
future. Insights and value assessment might be less clear or detailed if using
analyses that treat network power flows and constraints more simplistically, or
that do not integrate market and network dynamics.
In this example
(Figure 1), loads are located at four of the six busses. Zone 2 (busses D and F)
is a “load pocket” having limited transmission access to low-cost generation. The
characteristics of the generators, loads and transmission lines are summarized
in Tables 1 and 2. Loads cycle daily, peaking in the summer and having an
annual load factor of about 60%. The load profile is slightly different for
Zones 1 and 2.
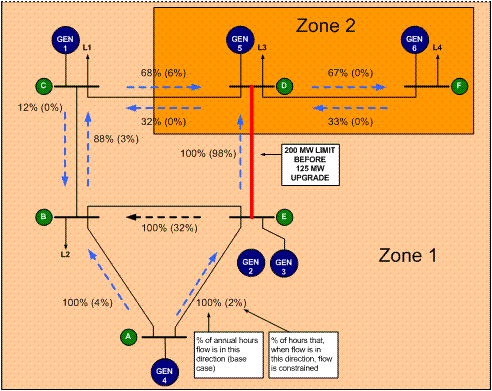
Figure 1. Example 7-Bus System, Indicating Base Case Flows and Congestion Prior to Transmission Upgrade
Key UPLAN simulation results examined included locational
electricity prices, generator revenues and net incomes, bus-specific consumer
costs, bus-specific unserved energy, and the hourly power flows, congestion and
congestion revenue rights (CRR) payments for each line. UPLAN provides the
capability to incorporate numerous N-1 transmission contingencies in generator
commitment and dispatch, as well as to dynamically integrate energy and
multiple ancillary services markets.[4]
While important for full-scale studies, these complexities were omitted from
(and are largely inappropriate for) this simple example. Noncompetitive bidding
could be examined, but was not.
Table 1. Generators and Loads (Base Case)
Bus
|
Generator or Load
I.D.
|
MW Capacity or Peak
Load
|
Generator Marginal
Cost (Bid), $/MWh
|
Average LMP, $/MWh <1>
|
Annual Operating
Hours
|
ZONE 1
|
|
|
|
|
|
A
|
Gen4
|
300
|
$33
|
$35.25
|
8617
|
B
|
L2
|
280
|
--
|
$42.31
|
--
|
C
|
Gen1
|
500
|
$42
|
$53.02
|
1640
|
C
|
L1
|
420
|
--
|
$43.51
|
--
|
E
|
Gen2
|
200
|
$32
|
$34.60
|
8729
|
E
|
Gen3
|
300
|
$35
|
$35.00
|
1584
|
ZONE 2
|
|
|
|
|
|
D
|
Gen5
|
350
|
$40
|
$49.67
|
7997
|
D
|
L3
|
800
|
--
|
$51.30
|
--
|
F
|
Gen6
|
200
|
$50
|
$55.84
|
3521
|
F
|
L4
|
200
|
--
|
$51.42
|
--
|
|
|
|
|
|
|
<1> Averaged over 8760 hours
(load-weighted) for loads, hours of operation for generators.
|
Table 2. Seven-Line Transmission Network
Line Name
|
Maximum Capacity
(MW)
|
Transmission Loss
(%)
|
Resistance
(pu)
|
Reactance (pu)
|
A2B
|
197
|
0.561
|
0.0025
|
0.0141
|
C2B
|
137
|
1.701
|
0.0010
|
0.0054
|
C2D
|
195
|
0.516
|
0.0030
|
0.014 9
|
E2D
|
200
|
0.945
|
0.0020
|
0.0125
|
E2A
|
154
|
0.135
|
0.0006
|
0.0032
|
D2F
|
174
|
1.751
|
0.0060
|
0.0320
|
B2E
|
199
|
0.647
|
0.0020
|
0.0120
|
The example system was simulated for one 8760-hour year
under three different generation-load cases: Base Case, Add Generation case and
Add Load case (described below). The impact of upgrading line E2D’s transmission
capability from 200 MW to 400 MW was examined under each generation-load case.
This represents a snapshot of one year, whereas actual studies would likely
consider several time horizons.
Base Case without Transmission Upgrade
As expected, power flow direction over 8760 hours of the
year is primarily towards the load buses and especially towards the “load
pocket” (Zone 2, busses D and F in Figure 1). The most frequently binding constraints
are on line B2E (reverse direction) and, especially on line E2D, both carrying power
out of generation-rich areas. Competitive marginal cost-based generator
bidding combined with transmission constraints produces locational marginal
prices (LMP) that vary substantially from bus to bus (Table 1), with load
pocket consumers experiencing high prices. As a consequence, the lowest-cost
generator in the load pocket (Generator 5) achieves a high net income.
Outside of the load pocket, high-cost Generator 1 operates
intermittently, but is sustained financially by occasional high LMP at its
location (Table 1), due to the way that transmission constraints affect network-wide
generator dispatch (discussed below). The lowest-cost generators 2 and 4 (buses
A and E) operate virtually all of the time, and their energy market income reflects
their infra-marginal costs. Higher cost Generator 3 has the misfortune of being
located in a “generation pocket” with limited access to loads, thus being on
the margin when it operates. To remain viable it might have to achieve
significant capacity or ancillary services revenues, and/or benefit from
generators bidding above marginal costs, none of which was considered in this example.[5]
Table 1 illustrates how LMP can exceed the bid of the
highest-bidding generator, in this case $50/MWh. This occurs often in the load
pocket and also affects Generator 1 at bus C, which runs when there are high
prices and congestion. The explanation is that the LMP at a bus represents the
incremental system cost for serving an increment of load at the
bus. With network constraints this may require not only ramping up some
generators but also ramping down others, so that the incremental cost can
exceed the cost of simply ramping up the marginal generator. Such interdependencies
are addressed by realistic power flow models, and will generally be most
pronounced where the network is substantially non-radial and experiencing
congestion. Transmission upgrades can then have widespread and sometimes
unexpected effects on economic efficiency.
Value of Transmission Investment (Base Case)
The
transmission investment consisting of upgrading line E2D from 200 MW to 400 MW
capacity was valued based on four components of value:
- consumer surplus, based on reduction
in annual electric energy payments by consumers overall and at each bus,[6]
- reliability of service to loads
overall and at each bus,
- producer surplus based on generator
net income overall and for each generator[7]
- congestion payments received by
transmission owners and/or holders of congestion revenue rights (CRRs),
overall and by transmission line.
Reliability of service can be valued
in different ways. For a short time horizon with no warning or opportunity to
mitigate, the value of reliability may be very high, having typically been estimated
on the order of thousands of dollars per MWh of unserved energy.[8]
However, our example assumes a longer “investment” time horizon, and calculates
the reliability value of a transmission upgrade based on the avoided cost of alternative
measures for maintaining reliability. The cost of such alternative measures is
assumed to equal a capacity cost of $80 per kW-year per peak kW of unserved
demand eliminated, plus an energy cost of $50 per MWh of unserved energy eliminated.
This is very roughly equivalent to the cost of a new, advanced combustion
turbine.
Under a locational pricing system
incorporating congestion revenue rights (CRRs), congestion payments associated
with any transmission line represent the difference between what consumers pay
for electricity imported over that line, versus what the exporting producers
receive. This difference occurs because consumers pay based on their locational
price while producers are paid based on their locational price, and these
locational prices differ when congestion restricts optimal power flows.[9]
Thus, when transmission constraints lead to inefficient generator dispatch and
elevated consumer payments, some of these elevated payments go to generators,
and some go to holders of CRRs (who in some instances may be the transmission
owners).[10]
Not surprisingly, in the Base
Case, consumer energy cost savings dominate the transmission upgrade benefit
calculations, so that the $56.2 million reduction in annual consumer energy
payments greatly exceeds in magnitude the income losses for generators and CRR holders
(Table 3). The upgrade eliminates unserved energy, but the consumer energy cost
benefit is substantially greater than the reliability benefit. However,
including the reliability benefit significantly increases the net benefit
(after subtracting producer and transmission owner disbenefits) by about 50%.
The overall result suggests a stakeholder
class distributional issue in that consumers gain and both generators and CRR
holders lose. Where load serving entities own the generation and CRRs, this distributional
issue may recede, if generator net incomes and congestion revenues are flowed
back to consumers to offset revenue obligations.
Table 3. Breakdown of
Transmission Upgrade Benefits Under Three Generation-Load Cases
|
Base Case
|
Add Generation
|
Add Load
|
Consumer Energy Cost Benefit
|
|
|
|
Zone 1
|
$8.8M
|
($3.5M)
|
$37.1M
|
Zone 2
|
$47.4M
|
$32.0M
|
$83.0M
|
Total
|
$56.2M
|
$28.4M
|
$120.1M
|
Reliability Benefit (~all in Zone 2)
|
$12.0M
|
$1.9M
|
$16.8M
|
Producer Surplus Benefit
|
($35.1M)
|
($20.6M)
|
($82.5M)
|
Congestion Revenues Benefit
|
($1.9M)
|
($1.0M)
|
($8.6M)
|
Total Benefit
|
$31.1M
|
$8.7M
|
$45.8M
|
We now consider how transmission
investment benefits are distributed among stakeholders, particularly the two
load zones and six generators. Of course, some generators could be affiliated with
other generators or with load serving entities. On the other hand, there could
be more complex stakeholder interests such as based on contracts that hedge and
reallocate risks and benefits. In any event, detailed and realistic modeling of
the network, the market and their interaction is required to distinguish
impacts on different stakeholders, especially in more complex real-world
situations.
Under the Base Case, all consumers
(all load buses) benefit from the upgrade, although those in the load pocket benefit
the most, with their energy payments dropping by more than 17%, versus a 5.5%
for other consumers. Realistic network simulation captures the strong
interrelationship among network elements and generators, including the way that
the transmission upgrade on line E2D provides greater flexibility for operating
the overall system, not merely for moving power into the load
pocket. This explains how consumers outside of the load pocket can benefit from
the upgrade. Before (but not after) the upgrade, overall network constraints caused
the LMP at bus C outside the load pocket to occasionally reach high levels
above $50/MWh, due to sometimes having to adjust dispatch across the network in
order to serve the last increment of load at bus C.[11]
For generators, impacts of the
transmission upgrade are more dramatic and varied (Figure 2). Due to more
flexible and less constrained system operation after the upgrade, there is a large
reduction in prices in the load pocket, shrinking generator 5’s formerly
generous operating margins, and driving generator 6’s energy market margin essentially
to zero (perhaps requiring capacity, ancillary services or RMR payments to sustain
the generator). Generator 1 experiences a similar effect, since after the
upgrade it is able to operate more hours but at reduced prices, because the
upgrade eliminates power flow constraints that occasionally produced high
prices at Generator 1’s location.
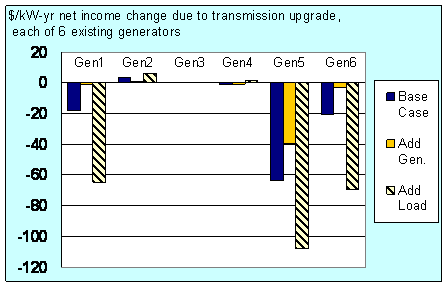
Figure 2: Changes in Generator Net Income Due to Transmission Upgrade
These generator outcomes
illustrate the substantial income loss faced by some generators due to
transmission upgrades in competitive markets having locational pricing for
generators. This raises questions of whether such generators are entitled to
any of their original profit margin, whether capacity markets, RMR or other
arrangements could keep them viable, or finally, whether it is simply
acceptable and economic for them to be replaced. These generators’ owners would
likely resist the transmission upgrade, and the assessment process might need
to consider stranded or retired generation scenarios.
As indicated in Table 3, change in overall congestion
revenues represents a relatively minor outcome of the transmission upgrade. The
disaggregated results are more interesting, in that the upgrade reduces
congestion and congestion revenues for line E2D and to a lesser extent for the
other Zone 2 import line C2D, but actually increases congestion on lines B2E
and C2D. This illustrates an important phenomenon in transmission planning:
investing to relax a binding constraint somewhere on an interconnected network
will facilitate economic power flows up to the point that some other
constraint(s) become binding. One recent transmission planning study using
UPLAN concluded that “although there are often hidden layers that could
potentially be binding constraints, they have no impact on the generation
dispatch until the outermost layer [the initial constraint] is removed…. a
power flow analysis is not capable of determining which of several
contingency/overload pairs is the most binding constraint; however, UPLAN is
capable of identifying which one is most binding (costly)…”[12]
In our example, the upgrade
actually increased projected total line-hours of congestion (one line congested
for one hour = one line-hour), but reduced the cost of congestion, as the
upgrade was intentionally focused on the economically most burdensome constraint.
Since the investment decreased congestion revenues for the upgraded line (by reducing
price differentials across the line), receipt of congestion revenue rights
(CRR) for the line might not be a good motivator for transmission investment.
Alternative Futures
UPLAN’s network-market simulations can be used to evaluate
numerous uncertainties, including automated volatility analysis that converts
input probability distributions for key drivers into probability distributions
for such results as prices and asset values. Here, we simply examine two
discrete alternative scenarios regarding generation and load, “Add Generation”
with a new, low-cost 125 MW generator added in the load pocket at bus D, and
“Add Load” with the load at bus D increased by 10%. Actual transmission
investment valuation would typically consider a much wider range of
uncertainties.
Under “Add Generation”, net societal benefit of the upgrade
is about $22 million/year (over 70%) less than in the Base Case, due to lower
prices and reliability problems in the load pocket, to start with (Table 3).
However, under “Add Load”, the societal value of the upgrade is about $15
million per year (about 45%) higher than under the Base Case, due to starting
with more constrained supply and higher prices.
The net benefits can be disaggregated. Under “Add
Generation” the load pocket experiences lower energy costs and reliability
problems to start with, so that the transmission upgrade produces $28
million/year less consumer energy cost benefit and $10 million/year less
consumer reliability benefit, compared to benefits under the Base Case (Table
3). However, “Add Generation” also starts with lower generator incomes and
congestion revenues, so that the upgrade has a $15 million/year lower impact in
reducing producer income and a $1 million/year lower impact in reducing CRR revenues, compared to the Base Case.
Under “Add Load” the opposite occurs. Starting from higher
levels of congestion-driven prices and producer surplus, the transmission upgrade
has greater impacts. It produces $64 million/year greater consumer energy cost
benefit than it did under the Base Case, and $5 million/year greater
reliability benefit. However, these increased benefits are mostly offset by a
producer surplus decline (due to the upgrade) $47 million/year higher than the
decline projected under the Base Case, and a CRR revenue decline $7
million/year higher. It could (and likely would) be debated how loss of high
congestion-driven revenues should be treated in the “societal benefit”
calculation. In any event, such revenue effects illustrate how even competitive
(non-strategic, not exercising market power) generator bidding can produce high
returns when there are substantial transmission constraints.
Finally, note that under the Add Load case’s constrained
supply conditions, even consumers outside of the load pocket benefit
considerably from the transmission upgrade, since pre-upgrade network operation
was so severely restricted as to even elevate some consumer prices outside of
the load pocket. However, under the Add Generation case’s more relaxed supply
conditions, consumers outside of the load pocket are very slightly harmed by
the upgrade, because the main impact of the upgrade is simply to permit export
of additional lower-cost energy out of Zone 1 into the load pocket (Zone 2).
Among the generators, impacts of the transmission upgrade
fall upon the three generators originally benefiting the most from transmission
constraints (Figure 3). Tighter supply conditions under “Add Load” produce the
greatest network constraints and the greatest pre-upgrade revenues for these
generators, as well as the greatest upgrade-caused income declines. Without the
upgrade, price run-ups resulting from transmission constraints clearly aid
these generators’ financial survival. What is less clear is how to value (and
what to do about) their possibly fatal economic losses if the upgrade is
implemented. The other generators see little economic impact from the upgrade.
Conclusions
The wide distribution, longevity and uncertainty of
transmission benefits help make it unwise to rely on “the market” for
transmission investment. For valuing and selecting such investments, a
realistic, integrated network- and market-wide “big picture” assessment is very
helpful, in order to capture broad system-wide benefits while also revealing
the distribution of stakeholder impacts. Why praise strategic transmission
planning unless we are willing to apply it in actual investment decisions?
This example illustrates how the value of transmission
upgrades can be counter intuitively large and widespread, using electrically
realistic integration of network power flows and electricity market dynamics,
even without the further complexities of security-constrained commitment and
dispatch, or strategic bidding. Furthermore, consumers will generally be the
main beneficiaries of network upgrades, especially if stressed supply
conditions might occur, and even consumers physically removed from the upgrade
itself can benefit. Furthermore, transmission investment may show especially
high value to consumers under low-probability, high-impact events such as major
outages or disruptions, which should be considered in transmission valuation.
On the other hand, there may be some losers, especially among generators
previously benefiting from congestion. An integrated, realistic and
sufficiently detailed analysis provides the best basis for characterizing,
evaluating and discussing the potential stakeholder gains and losses.
Bios and Contact Information
Dr. Rajat Deb is
the founding president of LCG Consulting, Los Altos, California and chief
architect of the pioneering UPLAN program. He has more than 30 years experience
in energy industry and academia and has contributed extensively to both the
theory and practice in the energy field. Dr. Keith D. White has
consulted on energy matters for 25 years, focusing on technical, economic,
environmental and policy aspects of electricity supply, as well as transmission
planning and market design. Other staff members of LCG have contributed to the
model simulation and provided valuable comments and suggestions for this
paper. Dr. Deb can be reached at deb@energyonline.com
or 650-962-9670.